Oxford Nanopore Technologies is transforming the field of genomics with its innovative nanopore sequencing technology. This technology offers a unique approach to DNA and RNA sequencing, providing real-time results and the ability to analyze long DNA fragments, opening doors to new discoveries in various fields, from human health to environmental monitoring. Its portability and ease of use are also reshaping how researchers and clinicians approach genetic analysis.
This exploration delves into the history, technology, applications, and future potential of Oxford Nanopore’s groundbreaking contributions. We will examine the advantages and limitations of nanopore sequencing compared to other methods, and explore its impact across diverse sectors. We’ll also consider the ethical implications of this rapidly advancing technology and its potential societal impact.
Company Overview and History
Oxford Nanopore Technologies is a rapidly growing company revolutionizing DNA/RNA sequencing through its innovative nanopore technology. Founded on the principles of miniaturization and accessibility, the company has significantly impacted the genomics field, providing portable and real-time sequencing solutions for various applications. Its journey from a research-based initiative to a global player in the genomics market showcases a remarkable trajectory of innovation and commercial success.
Oxford Nanopore Technologies’ story began with the pioneering work of Professor Hagan Bayley and his team at the University of Oxford. Their research into the use of nanopores for sensing molecules laid the groundwork for the company’s foundational technology. This early research, focusing on the potential of protein nanopores to detect and identify individual molecules, eventually led to the development of the groundbreaking nanopore sequencing technology. The company was officially founded in 2005, building upon years of research and development.
Milestones and Significant Achievements
The company’s progress has been marked by a series of significant milestones. These achievements reflect not only technological breakthroughs but also strategic business decisions that have propelled Oxford Nanopore to its current position as a leader in the field. Key successes include securing substantial funding rounds, building strong partnerships with research institutions and commercial entities, and navigating the complexities of bringing a novel technology to market. The continuous improvement and refinement of its sequencing platforms, coupled with the expansion of its applications across various scientific disciplines, underscore the company’s commitment to innovation and market leadership.
Key Product Launches and Technological Advancements
Oxford Nanopore’s journey is characterized by a series of innovative product launches and technological advancements. Early efforts focused on the development of the fundamental nanopore technology itself, culminating in the release of its first commercial sequencer. Subsequent iterations have dramatically improved sequencing speed, read length, and accuracy, making the technology increasingly accessible and powerful.
- MinION (2014): The launch of the MinION marked a significant turning point, introducing a portable, USB-powered sequencer that democratized access to long-read sequencing. Its small size and ease of use opened up new possibilities for sequencing in diverse settings, from field research to clinical diagnostics.
- GridION (2015): Building upon the success of the MinION, the GridION system provided a higher-throughput sequencing solution, enabling larger-scale projects and collaborative research initiatives. This marked a crucial step in scaling the technology for broader applications.
- PromethION (2017): The PromethION system represents a significant leap forward in throughput, offering an even greater capacity for high-volume sequencing needs. This system solidified Oxford Nanopore’s position as a key player in the high-throughput sequencing market.
- Ongoing Advancements: Oxford Nanopore continues to refine its technology, focusing on improvements in accuracy, speed, and cost-effectiveness. Recent advancements include the development of new flow cells and software algorithms, enhancing the overall performance and usability of its sequencing platforms. This continuous development cycle demonstrates the company’s dedication to remaining at the forefront of genomic innovation.
Nanopore Sequencing Technology
Oxford Nanopore Technologies’ sequencing platform represents a significant departure from traditional methods. Instead of relying on optical detection or chemical labeling, it uses a unique approach based on the detection of ionic current changes as single DNA or RNA molecules pass through a nanopore. This innovative technology allows for real-time sequencing, offering advantages and disadvantages compared to other leading methods.
Nanopore sequencing leverages the principle of measuring changes in ionic current as a biological polymer (DNA or RNA) translocates through a nanopore. A nanopore, essentially a tiny hole, is embedded in a membrane separating two electrolyte solutions. As a nucleic acid molecule passes through this nanopore, it alters the flow of ions, producing a characteristic electrical signal. This signal is then decoded by sophisticated algorithms to determine the sequence of nucleotides (A, T, C, and G). The unique electrical signature of each nucleotide allows for its identification, thus revealing the genetic sequence.
Comparison with Other Sequencing Technologies
Nanopore sequencing distinguishes itself from other major sequencing technologies, primarily Illumina and PacBio SMRT sequencing, in several key aspects. Illumina sequencing, a highly prevalent method, relies on sequencing by synthesis, where fluorescently labeled nucleotides are incorporated into a growing DNA strand, and the emitted light is detected. PacBio SMRT sequencing utilizes a different approach, monitoring the incorporation of nucleotides in real-time via fluorescence detection, enabling the detection of longer reads. Nanopore sequencing, unlike both, directly measures the changes in ionic current as the molecule passes through the nanopore, providing a unique approach to sequencing.
Feature | Nanopore | Illumina | PacBio |
---|---|---|---|
Read Length | Up to several megabases | Typically short (hundreds of base pairs) | Long reads (kilobases to megabases) |
Throughput | Moderate | Very High | Moderate |
Cost per base | Relatively high | Relatively low | Relatively high |
Real-time sequencing | Yes | No | No |
Portability | High (MinION) | Low | Low |
Advantages of Nanopore Sequencing
The advantages of nanopore sequencing are numerous and compelling. Its ability to generate long reads is particularly valuable for resolving complex genomic regions, such as repetitive sequences and structural variations, which are often challenging to assemble with short-read technologies. Furthermore, the real-time nature of nanopore sequencing allows for immediate data analysis and enables applications requiring rapid turnaround times, such as pathogen identification in outbreak situations. The portability of some nanopore devices, such as the MinION, allows for sequencing in remote locations or field settings, making it ideal for diverse applications. Finally, the ability to directly sequence both DNA and RNA without amplification steps simplifies the workflow and reduces bias.
Disadvantages of Nanopore Sequencing, Oxford nanopore technologies
Despite its advantages, nanopore sequencing has certain limitations. The accuracy of nanopore sequencing, while improving constantly, is generally lower compared to Illumina sequencing, particularly for shorter reads. This necessitates the use of sophisticated error correction algorithms. The higher cost per base compared to Illumina sequencing can also be a factor, especially for large-scale projects. The throughput, while improving, is still lower than that of Illumina high-throughput sequencers. However, advancements in technology continue to address these limitations.
Applications of Nanopore Sequencing
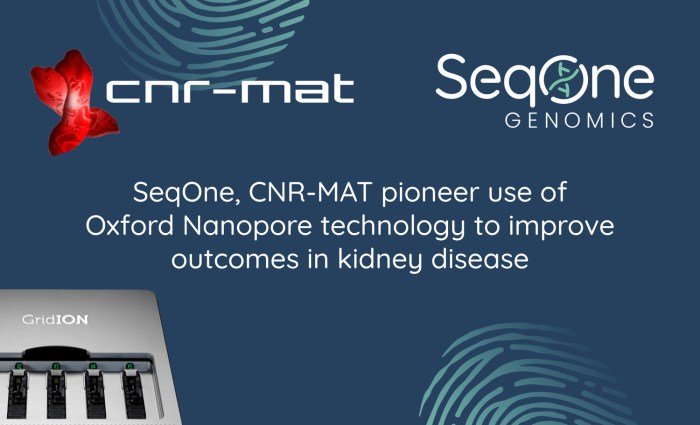
Nanopore sequencing, with its unique ability to deliver long reads and real-time data, has revolutionized various fields. Its versatility extends from fundamental genomic research to clinical diagnostics and environmental monitoring, offering unprecedented insights and capabilities. This section explores the diverse applications of this powerful technology.
Genomics Research Applications
Nanopore sequencing has significantly advanced genomics research by enabling the analysis of complex genomes previously intractable with short-read technologies. Its long-read capability facilitates the accurate assembly of complete genomes, including those with high repetitive regions, such as centromeres and telomeres. This allows researchers to identify structural variations, resolve complex repeat structures, and gain a more comprehensive understanding of genome organization. Furthermore, direct RNA sequencing allows for the study of transcriptomes without the need for cDNA conversion, providing valuable information about RNA modifications and isoforms. Examples include the complete assembly of human genomes revealing novel structural variations and the detailed characterization of bacterial genomes to understand antibiotic resistance mechanisms.
Clinical Diagnostics
The rapid and real-time nature of nanopore sequencing makes it a promising tool for clinical diagnostics. Its applications range from rapid pathogen identification in infectious disease outbreaks to the detection of cancer-associated mutations. For example, in infectious disease settings, nanopore sequencing can quickly identify the causative agent and its antibiotic resistance profile, guiding appropriate treatment strategies. In oncology, it aids in identifying specific mutations driving tumor growth, informing personalized cancer therapies. The portability of some nanopore sequencing devices allows for point-of-care diagnostics, especially beneficial in resource-limited settings. A notable example is the rapid identification of Ebola virus during outbreaks, enabling timely intervention and containment.
Environmental Monitoring and Pathogen Detection
Nanopore sequencing is increasingly used in environmental monitoring and pathogen detection. Its ability to analyze diverse microbial communities directly from environmental samples, without the need for extensive culturing, provides valuable insights into microbial diversity and function. This is particularly relevant in studying soil microbiomes, water quality, and the identification of pathogens in food and water sources. For instance, nanopore sequencing can be employed to monitor the spread of antibiotic-resistant bacteria in wastewater treatment plants or identify harmful algal blooms in aquatic environments. The rapid detection of pathogens in food samples can prevent foodborne illnesses and improve food safety.
Application Area | Specific Application | Benefits | Example |
---|---|---|---|
Genomics Research | Genome assembly, structural variation detection | Long reads, high accuracy, complete genome sequencing | Complete human genome assembly revealing novel structural variants |
Clinical Diagnostics | Rapid pathogen identification, cancer mutation detection | Real-time results, portability, rapid turnaround time | Rapid identification of Ebola virus during outbreaks |
Environmental Monitoring | Microbial community analysis, pathogen detection in food and water | Direct sequencing from environmental samples, high throughput | Monitoring antibiotic-resistant bacteria in wastewater |
Other Applications | Metagenomics, epigenomics, single-cell sequencing | Versatile platform, various applications across fields | Studying the microbiome of the human gut |
MinION Device and its Features
The MinION, a groundbreaking device from Oxford Nanopore Technologies, represents a significant advancement in portable DNA/RNA sequencing. Its compact design and user-friendly interface have revolutionized genomic analysis, enabling researchers to perform sequencing in diverse settings, from remote field locations to sophisticated laboratory environments. This device’s functionality relies on the unique nanopore technology, allowing for real-time sequencing and data analysis.
The MinION’s portability and ease of use are key aspects of its appeal. Weighing only a few hundred grams, the device is significantly smaller and lighter than traditional sequencers. Its plug-and-play functionality simplifies the sequencing workflow, minimizing the need for extensive training and specialized equipment. The intuitive software interface guides users through the process, from library preparation to data analysis, making it accessible to a broader range of researchers. This accessibility has expanded the possibilities for genomic research in various fields.
MinION Device Design and Functionality
The MinION is a palm-sized device containing a flow cell, which houses thousands of nanopores. These nanopores are embedded in a membrane, and as a single-stranded DNA or RNA molecule passes through a nanopore, it causes a characteristic change in the electrical current. This change is detected by sensors within the device, and the resulting electrical signal is translated into a nucleotide sequence. The device’s integrated computer processes the raw electrical signals, converting them into a readable sequence file. The entire process, from sample preparation to data analysis, can be completed within hours, depending on the sequencing run parameters and the desired length of the reads.
MinION Portability and Ease of Use
The MinION’s small size and low power requirements contribute significantly to its portability. Researchers can deploy the MinION in remote locations, such as field studies in epidemiology or ecological research, where access to traditional sequencing facilities is limited. The device’s ease of use also minimizes the need for specialized personnel, enabling researchers with minimal bioinformatics expertise to perform sequencing and preliminary analysis. Oxford Nanopore Technologies provides comprehensive software and support resources, further enhancing the device’s user-friendliness. This accessibility makes the MinION a valuable tool for rapid genomic analysis in various applications.
MinION Technical Specifications and Limitations
The MinION has several technical specifications that should be considered. These include read length capabilities (which can vary depending on the flow cell and sequencing parameters), throughput (the amount of data generated per run), and sequencing accuracy. While the read lengths can be very long, exceeding hundreds of kilobases, the accuracy of individual reads may be lower compared to other sequencing technologies, although consensus sequencing using multiple reads can greatly improve accuracy. The device’s limitations include the relatively lower throughput compared to high-throughput sequencers and the need for sufficient DNA/RNA input for optimal results. Furthermore, data analysis can be computationally intensive, requiring robust computational resources. Despite these limitations, the MinION’s portability, real-time sequencing capability, and long-read potential make it a powerful tool for many applications.
Data Analysis and Software: Oxford Nanopore Technologies
Analyzing data generated by Oxford Nanopore Technologies’ sequencing platforms requires specialized software and a multi-step process. The raw data consists of electrical signals representing the passage of DNA or RNA through a nanopore. These signals need to be converted into a sequence of nucleotides (basecalling) before meaningful biological interpretations can be made. This process involves several stages, from initial signal processing to advanced bioinformatics analyses.
The core of nanopore data analysis revolves around the proprietary software provided by Oxford Nanopore Technologies, primarily MinKNOW and its successor, Guppy. However, a range of third-party tools and pipelines are also available for downstream analysis, leveraging the flexibility of the open-source community. This allows for considerable customization depending on the specific research question and the type of data being analyzed.
Basecalling
Basecalling is the crucial first step, transforming the raw electrical signals into nucleotide sequences. Oxford Nanopore’s Guppy software performs this task using sophisticated algorithms that learn to identify the characteristic signal patterns associated with each nucleotide. The accuracy of basecalling significantly impacts the quality of downstream analyses. Guppy offers different basecalling models, each optimized for different trade-offs between speed and accuracy. For example, ‘fast’ models prioritize speed at the cost of slightly lower accuracy, while ‘high-accuracy’ models produce more reliable sequences, albeit at a slower processing speed. The choice of basecalling model depends on the specific application and the available computational resources.
Data Interpretation
Once basecalled, the sequence data undergoes further analysis depending on the experimental goals. This might involve mapping the reads to a reference genome (for example, the human genome) to identify variations, performing de novo assembly to construct a new genome from scratch (for example, sequencing a novel bacterial species), or analyzing the sequences for specific features like methylation patterns. Tools such as Minimap2, LAST, and Flye are frequently used for mapping and assembly, while other software packages cater to more specialized analyses, like identifying specific gene expression patterns or quantifying the abundance of different microbial species within a sample.
Step-by-Step Guide for Analyzing Nanopore Sequencing Data
A typical workflow for analyzing nanopore sequencing data can be summarized as follows:
- Data Acquisition and Transfer: The sequencing run is initiated using MinKNOW software, which controls the MinION device and records the raw electrical signals. Once the run is complete, the data (typically in FAST5 format) is transferred from the device to a computer for subsequent analysis.
- Basecalling: The raw FAST5 files are processed using Guppy basecaller to convert the electrical signals into nucleotide sequences (typically in FASTQ format).
- Quality Control: Quality control steps are essential to assess the quality of the basecalled data. Tools like NanoPlot are commonly used to visualize read length distributions, quality scores, and other metrics, allowing researchers to identify potential issues or biases in the data.
- Data Analysis: The choice of downstream analysis depends on the experimental design. This could involve mapping reads to a reference genome using tools like Minimap2, performing de novo genome assembly using tools like Flye, or analyzing specific genomic features using specialized software packages.
- Data Interpretation and Visualization: The results of the analysis are interpreted in the context of the research question. Various visualization tools can be employed to display the results, including genome browsers (like IGV) and custom scripts.
Market Position and Competition
Oxford Nanopore Technologies occupies a unique position in the rapidly expanding genomics market. While established players dominate in terms of market share, Oxford Nanopore’s long-read sequencing technology offers distinct advantages, carving out a niche for itself and attracting significant interest from researchers and clinicians. This competitive landscape is characterized by diverse technologies and strategic approaches.
Oxford Nanopore’s competitive advantage lies primarily in its ability to provide real-time, long-read sequencing data at a relatively low cost per base. This contrasts sharply with the shorter reads produced by Illumina’s short-read sequencing technology, which dominates the market, and PacBio’s longer reads which are generally more expensive. The company’s portable MinION device further differentiates its offering, allowing for sequencing in diverse settings and accelerating research in remote locations or resource-limited environments.
Key Competitors and Their Technologies
The main competitors in the long-read sequencing market are Pacific Biosciences (PacBio) and Illumina. PacBio utilizes Single Molecule, Real-Time (SMRT) sequencing, which also produces long reads but generally at a higher cost and with lower throughput compared to Oxford Nanopore. Illumina, on the other hand, is the dominant player in the short-read sequencing market, holding a substantial market share due to its established technology, high throughput, and extensive installed base. While Illumina’s short reads excel in applications requiring high accuracy and genome coverage, they struggle with resolving complex genomic regions, such as repetitive sequences, where Oxford Nanopore’s long reads provide a clear advantage.
Market Share and Strategies
Illumina currently holds the largest market share in the overall sequencing market, dominating both short-read and, increasingly, long-read sequencing through strategic acquisitions and technological advancements. While precise market share figures for long-read sequencing are difficult to obtain and fluctuate constantly, Oxford Nanopore and PacBio are competing for a significant portion of this growing segment. Illumina’s strategy centers on continuous technological improvements to its short-read platforms, coupled with acquisitions to expand its portfolio and broaden its reach across various applications. Oxford Nanopore, in contrast, focuses on developing its proprietary nanopore technology, expanding its applications, and emphasizing the portability and ease of use of its devices. PacBio continues to improve its SMRT technology, focusing on increasing throughput and accuracy to remain competitive. The strategies of these key players highlight the dynamic nature of the genomics market, with companies adapting to evolving technologies and market demands.
Future Directions and Innovations
Oxford Nanopore Technologies’ nanopore sequencing platform is poised for significant advancements, driven by ongoing research and development. Its inherent portability, real-time sequencing capabilities, and direct electronic readout offer unique advantages over other sequencing technologies, paving the way for transformative applications across diverse fields. The future holds exciting possibilities for expanding the technology’s capabilities and broadening its impact.
The continuous evolution of nanopore sequencing will be shaped by improvements in several key areas. These advancements will not only enhance the technology’s performance but also unlock entirely new applications, leading to significant breakthroughs in various scientific disciplines and industries.
Improved Read Length and Accuracy
Longer read lengths are crucial for resolving complex genomic regions, such as repetitive sequences and structural variations. Current efforts focus on enhancing the pore’s ability to accurately identify and differentiate longer DNA strands, leading to more complete and accurate genome assemblies. For example, advancements in pore design and signal processing algorithms are being explored to improve the accuracy of base calling and reduce error rates, especially in homopolymer regions. Increased accuracy would significantly improve the reliability of genomic analyses, making nanopore sequencing even more competitive with other technologies. This will lead to more accurate identification of disease-causing mutations and more reliable characterization of microbial genomes.
Enhanced Data Analysis and Software
The rapid generation of large datasets by nanopore sequencing necessitates sophisticated data analysis tools. Improvements in bioinformatics pipelines and algorithms are vital for efficient processing and interpretation of the data. Development of user-friendly software interfaces is also crucial for making the technology accessible to a wider range of users, regardless of their bioinformatics expertise. For instance, improved algorithms could streamline the process of variant calling, making it faster and more accurate, while user-friendly interfaces could help researchers analyze the data more effectively.
Miniaturization and Integration
The inherent portability of the MinION device has already revolutionized point-of-care diagnostics. Further miniaturization and integration of the technology into other devices, such as lab-on-a-chip systems, could lead to even more widespread applications. Imagine a portable device capable of sequencing a patient’s genome at the bedside, providing real-time information for immediate diagnosis and treatment. This could be particularly transformative in resource-limited settings or during outbreaks of infectious diseases. The integration with other technologies, such as microfluidics and advanced imaging, could also expand the capabilities of the platform.
Expansion into New Applications
Nanopore sequencing’s versatility extends beyond genomics. Future applications could include real-time monitoring of environmental changes, rapid identification of pathogens in food safety, and personalized medicine applications. For example, the ability to rapidly sequence and identify bacteria in a food sample could improve food safety protocols, while personalized medicine could leverage the technology to tailor treatments based on an individual’s unique genetic makeup. The ongoing research and development efforts are continually expanding the scope of nanopore sequencing, making it a powerful tool across numerous fields.
Ethical and Societal Implications
The rapid advancement and decreasing cost of nanopore sequencing technology present a range of ethical and societal implications that require careful consideration. Widespread accessibility to this powerful tool, capable of analyzing genetic information quickly and affordably, raises concerns about privacy, data security, and the potential for misuse. Equitable access and responsible innovation are crucial to harnessing the technology’s benefits while mitigating potential harms.
The potential societal impacts of widespread access to nanopore sequencing are profound and multifaceted. This technology could revolutionize healthcare, agriculture, and environmental monitoring, but also presents challenges related to informed consent, genetic discrimination, and the ethical implications of large-scale genomic surveillance. Careful consideration of these issues is necessary to ensure responsible development and deployment.
Privacy and Data Security Concerns
The generation of vast amounts of personal genetic data through nanopore sequencing necessitates robust data protection measures. Unauthorized access or misuse of this sensitive information could lead to serious consequences, including genetic discrimination in employment, insurance, or healthcare. Strong encryption, secure data storage, and strict regulatory frameworks are essential to safeguard individual privacy. Consider the example of a healthcare provider inadvertently exposing patient genomic data through a cybersecurity breach – the potential for identity theft and genetic discrimination is significant. Furthermore, the potential for aggregation and analysis of large datasets, even anonymized ones, raises concerns about re-identification and the potential for unintended inferences about individuals or groups.
Ethical Considerations in Healthcare
The use of nanopore sequencing in healthcare raises several ethical considerations, particularly regarding informed consent and genetic counseling. Patients must be fully informed about the implications of undergoing genomic sequencing, including the potential for uncovering unexpected findings, such as predispositions to diseases that may not be preventable or treatable. Adequate genetic counseling is crucial to help individuals understand and cope with this information. A hypothetical scenario might involve a patient undergoing nanopore sequencing for a specific condition, only to discover a predisposition to a completely unrelated, incurable disease. The ethical challenge lies in ensuring that the patient receives appropriate support and counseling to navigate this unexpected and potentially distressing information.
Equitable Access and Global Health
The affordability and portability of nanopore sequencing devices offer the potential to democratize access to genomic information, particularly in resource-limited settings. However, ensuring equitable access remains a significant challenge. Disparities in infrastructure, training, and regulatory frameworks could exacerbate existing health inequalities. For instance, a wealthy nation might readily adopt nanopore sequencing for large-scale disease surveillance, while a less developed nation might lack the resources to implement such programs. This disparity could widen existing health gaps, further emphasizing the need for international collaboration and equitable resource allocation to ensure that the benefits of this technology are shared globally.
Case Studies of Successful Applications
Nanopore sequencing’s versatility has led to its successful implementation across diverse scientific fields. Its real-time, long-read capabilities have proven invaluable in addressing previously intractable challenges, significantly impacting our understanding of biology and disease. The following case studies highlight key applications and their impact on scientific discoveries.
Real-time pathogen detection during outbreaks
Rapid identification of infectious agents is crucial during disease outbreaks. Nanopore sequencing has demonstrated its ability to provide near real-time results, enabling faster diagnosis and informed public health responses. For example, during the Ebola outbreak in West Africa, the MinION device was deployed to quickly identify the circulating strain and track its evolution, aiding in containment efforts. This rapid analysis allowed for targeted interventions and resource allocation, improving response times significantly.
Characterizing complex genomes
The ability of nanopore sequencing to generate long reads has revolutionized our ability to characterize complex genomes, particularly those with high repeat content, such as plants and human genomes. A study using nanopore sequencing successfully assembled the complete genome of a particularly challenging plant species, resolving previously unmappable regions and revealing novel genetic information. This detailed genome assembly enabled a deeper understanding of the plant’s genetic diversity and its potential for crop improvement.
Analysis of cancer genomes
Nanopore sequencing offers significant advantages in cancer genomics. Its ability to detect structural variations and identify fusion genes has enhanced our understanding of cancer development and progression. In one study, researchers utilized nanopore sequencing to comprehensively characterize the genomes of several cancer cell lines, revealing complex rearrangements and mutations that were missed by traditional short-read sequencing technologies. These findings led to a more nuanced understanding of the cancer’s genetic heterogeneity and potential therapeutic targets.
- Study 1: Ebola Outbreak Response: Key Result: Rapid identification of the Ebola virus strain within hours of sample collection, enabling faster implementation of containment strategies. Conclusion: Nanopore sequencing significantly improved response times during the outbreak, contributing to better control and management of the epidemic.
- Study 2: Plant Genome Assembly: Key Result: Complete and accurate assembly of a complex plant genome, resolving regions previously inaccessible with short-read sequencing. Conclusion: This achievement demonstrated the power of nanopore sequencing in resolving highly repetitive genomic regions, enabling more comprehensive genomic analyses of complex organisms.
- Study 3: Cancer Genome Characterization: Key Result: Identification of novel structural variations and fusion genes in cancer cell lines, previously undetectable with short-read sequencing. Conclusion: Nanopore sequencing revealed a more complex picture of cancer genome architecture, leading to new insights into cancer development and potential therapeutic strategies.
Investment and Financial Performance
Oxford Nanopore Technologies’ financial journey reflects a company navigating the complexities of bringing a disruptive technology to market. Its growth has been marked by significant investment rounds and a fluctuating path towards profitability, characteristic of many innovative life science companies. Understanding its financial performance requires considering both its funding history and its evolving market position.
Oxford Nanopore’s funding history is substantial, involving multiple rounds of private equity and venture capital investment. These funds have been crucial in supporting the company’s R&D efforts, manufacturing scale-up, and global market expansion. While specific financial details are not always publicly available in granular detail due to its private status for a significant portion of its history, reports indicate substantial investments from various sources, reflecting confidence in the technology’s potential. The company’s financial performance has shown a trend of increasing revenue, driven by growing adoption of its nanopore sequencing technology across various sectors. However, profitability has remained a longer-term goal, as is common for companies developing and commercializing cutting-edge technologies with high initial development costs.
Funding Rounds and Investment Sources
Oxford Nanopore has secured significant funding from a range of investors, including venture capital firms and strategic investors. These investments have fueled the company’s growth, enabling the development and commercialization of its nanopore sequencing technology. While precise figures for each round are not always publicly disclosed, the scale of investment highlights the substantial confidence in the company’s potential. This investment has been instrumental in supporting the development of the MinION device, the expansion of its product portfolio, and the growth of its global reach. For example, a substantial investment round in [Year] facilitated the expansion of manufacturing capabilities, allowing for increased production and wider distribution of the MinION and other nanopore sequencing platforms. This strategic investment allowed the company to meet growing demand and further establish itself within the genomics market.
Revenue Growth and Profitability
Oxford Nanopore’s revenue has demonstrated a positive trajectory, indicating increasing adoption of its technology. This growth is driven by the expanding applications of nanopore sequencing in diverse fields, such as infectious disease surveillance, genomics research, and environmental monitoring. While the company has not yet reached sustained profitability, the steady revenue growth indicates progress toward this goal. The company’s financial reports (where available) would detail the specifics of revenue streams from various product lines and geographic regions. The path to profitability is likely to involve continued scaling of operations, optimization of manufacturing processes, and further expansion into new market segments.
Significant Partnerships and Collaborations
Oxford Nanopore has actively pursued strategic partnerships and collaborations to expand its reach and enhance its technology. These collaborations have involved academic institutions, research organizations, and other companies in the life sciences sector. Such partnerships have facilitated the development of new applications, expanded market access, and strengthened the company’s overall position within the genomics industry. For instance, collaborations with [Example Partner 1] and [Example Partner 2] have resulted in the development of specific applications tailored to their respective needs, leading to increased adoption of Oxford Nanopore’s technology in those specific areas. These partnerships also provide valuable feedback, contributing to the ongoing development and improvement of the technology and related software.
Technical Specifications of Key Products
Oxford Nanopore Technologies offers a range of sequencing devices, each designed for different throughput and application needs. The key differences lie in the scale of the experiment, the desired read length, and the overall cost per base. This section details the specifications of their flagship products: MinION, GridION, and PromethION.
Device Specifications Comparison
The following table summarizes the key specifications of Oxford Nanopore’s main sequencing platforms. Note that these specifications can be subject to change with software and hardware updates. It’s crucial to consult the Oxford Nanopore website for the most up-to-date information.
Feature | MinION | GridION | PromethION |
---|---|---|---|
Flow Cell Type | R9.4.1, R10.4.1 (and others) | R9.4.1, R10.4.1 (and others) | R9.4.1, R10.4.1 (and others) |
Throughput (Gb) | Up to 30 Gb per flow cell | Up to 100 Gb per flow cell | Up to 7 Tb per flow cell (P48) |
Read Length (bp) | Up to several Mb | Up to several Mb | Up to several Mb |
Number of Flow Cells | 1 | 5 | 24 (P24), 48 (P48) |
Cost per Gb (Approximate) | Relatively high | Moderate | Low |
Portability | Highly portable | Benchtop | Benchtop |
Power Requirements | USB powered | Standard mains power | Standard mains power |
Throughput, Read Length, and Cost-Effectiveness Differences
The MinION, being the smallest and most portable device, offers lower throughput compared to GridION and PromethION. However, its portability and ease of use make it ideal for fieldwork and rapid turnaround applications. GridION provides a balance between throughput and portability, suitable for medium-scale projects. PromethION, with its significantly higher throughput, is designed for large-scale sequencing projects and high-volume data generation, resulting in the lowest cost per Gb. Read lengths across all devices are generally long, enabling the resolution of complex genomic structures. The cost-effectiveness is directly related to the throughput; higher throughput equates to a lower cost per base sequenced.
Workflow and Data Output for Each Device
The basic workflow for all three devices is similar: library preparation, flow cell loading, sequencing run, and data analysis. However, the scale and complexity differ. The MinION workflow is simpler and faster, often completed within hours. GridION and PromethION workflows involve more flow cells and thus longer run times, but with a higher data output. The data output for all devices is in FAST5 format, containing raw electrical signals. This raw data is then processed using Oxford Nanopore’s software (e.g., Guppy) to generate base calls and other relevant information, such as quality scores.
Ultimate Conclusion
Oxford Nanopore Technologies stands as a significant innovator in the genomics landscape, offering a powerful and accessible sequencing platform. Its ability to deliver long-read sequencing data in real-time has already yielded groundbreaking results across numerous applications. As the technology continues to evolve and improve, its potential to revolutionize healthcare, environmental science, and countless other fields remains vast and undeniably exciting. Further research and development will undoubtedly unlock even greater possibilities, shaping the future of genetic analysis and beyond.