Super Power Break Technology represents a hypothetical leap forward in engineering, promising transformative advancements across numerous sectors. This exploration delves into the feasibility, societal impact, and ethical considerations of such a revolutionary concept, examining its potential benefits and risks with a balanced perspective. We will analyze existing technologies that offer glimpses into the possibilities, while also acknowledging the significant hurdles that must be overcome before this vision becomes reality. The journey will encompass technological limitations, economic models, and environmental concerns, ultimately providing a comprehensive overview of this intriguing area of potential future development.
The core concept revolves around a technology capable of achieving a previously unimaginable level of efficiency or power within a specific application. This might involve breakthroughs in energy storage, materials science, or even entirely new paradigms of energy generation and transmission. We will consider various interpretations of what constitutes a “super power break,” comparing and contrasting different approaches and their implications.
Defining “Super Power Break Technology”
Super Power Break Technology (SPBT) refers to a hypothetical class of technologies designed to achieve exceptionally rapid and efficient deceleration or stopping of high-speed objects, far exceeding the capabilities of currently available braking systems. This encompasses not just physical braking, but also encompasses the rapid dissipation or redirection of kinetic energy in various systems, whether mechanical, electrical, or even biological. The core concept revolves around minimizing damage, maximizing safety, and achieving near-instantaneous halts, even under extreme conditions.
The defining characteristic of SPBT is its ability to handle forces and energy magnitudes far beyond the scope of conventional braking methods. This necessitates novel approaches to energy absorption and momentum management, potentially involving advanced materials, innovative designs, and sophisticated control systems. The ultimate goal is to achieve a “super power break”—a stopping capability that significantly surpasses current technological limitations.
Precursors to Super Power Break Technology
Several existing technologies serve as precursors to the concept of SPBT, demonstrating incremental steps toward achieving exceptionally rapid deceleration. These include advanced anti-lock braking systems (ABS) in automobiles, which prevent wheel lockup during hard braking, and the regenerative braking systems used in electric and hybrid vehicles, which recover kinetic energy during deceleration. Furthermore, the sophisticated landing gear and deceleration systems used in aircraft carriers for arresting the rapid landing of fighter jets showcase controlled high-energy dissipation. Magnetic levitation (Maglev) train technology, while not strictly a “braking” system, demonstrates exceptionally efficient deceleration through controlled magnetic fields. These examples highlight the ongoing pursuit of increasingly efficient and effective deceleration methods, paving the way for future SPBT developments.
Interpretations of a “Super Power Break”
Different interpretations of a “super power break” exist, depending on the application and context. In the context of transportation, it might involve near-instantaneous stops for high-speed vehicles, minimizing impact forces during collisions. In industrial settings, it could refer to the rapid deceleration of heavy machinery or robotic arms to prevent damage or accidents. For aerospace applications, it could involve rapidly slowing down spacecraft or projectiles upon atmospheric entry. The common thread is the extraordinary speed and efficiency of the deceleration process, far exceeding current technological capabilities. One interpretation might focus on the force reduction experienced by the decelerating object, while another might prioritize the distance required for complete deceleration. The definition of “super” is relative and context-dependent, ultimately defined by the specific application and the magnitude of improvement over existing technologies.
Technological Feasibility
The development of “Super Power Break Technology,” capable of instantaneously halting objects with significant kinetic energy, presents considerable technological hurdles. While the concept is captivating, the reality is far from straightforward, demanding breakthroughs across several scientific disciplines. Current limitations stem from our incomplete understanding of materials science at extreme stress levels, the limitations of energy storage and release mechanisms, and the challenges inherent in scaling such a system for practical applications.
Current technological limitations primarily involve the immense forces involved in abruptly stopping high-speed objects. The energy must be absorbed or redirected with incredible speed and efficiency, preventing catastrophic damage to the braking system itself and the surrounding environment. Existing braking systems, even those used in high-performance vehicles or aerospace applications, rely on gradual deceleration, making them unsuitable for the instantaneous stopping power envisioned in “Super Power Break Technology.” Furthermore, the precision required to halt an object without causing collateral damage is extraordinarily challenging.
Material Science Limitations and Potential Breakthroughs
The creation of a Super Power Break system necessitates materials with exceptional strength, durability, and energy absorption capabilities. Current materials, even advanced composites, would likely fail under the extreme stresses generated by rapidly halting a high-speed object. Potential breakthroughs might involve the development of novel metamaterials with tailored properties, or the advancement of nanomaterials with superior strength-to-weight ratios. Imagine a material capable of instantly dispersing the kinetic energy of a speeding bullet, transforming it into heat or other less harmful forms of energy, without structural failure. Research into advanced carbon nanotubes and graphene, alongside explorations into exotic materials like those found in advanced aerospace applications, offers potential pathways towards achieving this.
Energy Storage and Release Challenges
The rapid deployment of sufficient energy to instantaneously halt a moving object poses significant challenges in energy storage and release. The system would need to access and deploy a vast amount of energy in an incredibly short timeframe. Current battery technologies are unsuitable due to their limited power density and slow discharge rates. Potential breakthroughs might involve advancements in supercapacitors, which offer higher power density than batteries, or the development of entirely new energy storage methods, such as advanced flywheel systems or even controlled nuclear fusion reactions (though this is highly speculative at this point). For example, imagine a system utilizing a network of miniature, high-energy density supercapacitors that can instantly release their stored energy into a carefully designed energy dissipation mechanism.
Scalability and Cost-Effectiveness Considerations
Even with breakthroughs in materials science and energy storage, the scalability and cost-effectiveness of Super Power Break Technology remain significant obstacles. The size, weight, and energy requirements of such a system would likely be substantial, making it impractical for many applications. Furthermore, the manufacturing costs of materials with the required properties would be exceptionally high. For instance, imagine scaling the system from halting a small projectile to halting a high-speed train. The energy requirements would increase exponentially, alongside the size and complexity of the braking system. Cost-effective manufacturing processes would be critical for widespread adoption, potentially necessitating breakthroughs in additive manufacturing or other advanced production techniques.
Societal Impact
Super Power Break Technology, with its potential to revolutionize energy production and infrastructure, carries significant implications for society. Its widespread adoption could lead to profound changes, both positive and negative, impacting various aspects of human life and the environment. A careful consideration of these potential impacts is crucial for responsible development and deployment of this technology.
The societal impact of Super Power Break Technology is multifaceted and requires a nuanced understanding of its potential benefits and drawbacks. While the technology promises significant advancements, careful planning and regulation are essential to mitigate potential risks and ensure equitable distribution of its benefits.
Positive Societal Impacts
The potential positive societal impacts of Super Power Break Technology are extensive and could fundamentally reshape our world. Improved infrastructure, enhanced energy security, and economic growth are just some of the anticipated benefits.
Positive Impact | Description | Example |
---|---|---|
Improved Infrastructure | The technology’s ability to efficiently break down and recycle materials could lead to the creation of stronger, lighter, and more durable infrastructure components, reducing maintenance costs and increasing lifespan. This could also enable the construction of more resilient infrastructure capable of withstanding extreme weather events. | Imagine bridges and buildings constructed with materials that are significantly stronger and lighter due to the optimized recycling process enabled by Super Power Break Technology. This leads to less material use, lower construction costs, and higher resistance to earthquakes and storms. |
New Energy Sources | Super Power Break Technology could unlock new, efficient, and sustainable energy sources by enabling the cost-effective extraction of valuable materials from waste products. This could facilitate the transition to renewable energy systems and reduce reliance on fossil fuels. | Imagine a future where the waste products from agricultural processes are efficiently broken down to extract valuable elements used in the production of solar panels, significantly reducing the cost and environmental impact of solar energy production. |
Economic Growth | The development and deployment of this technology would create numerous job opportunities in research, manufacturing, and maintenance. Furthermore, the increased efficiency and productivity it offers across various industries could stimulate economic growth. | The development of Super Power Break Technology could spur the creation of new industries focused on material recycling and the production of advanced materials, leading to significant job creation and economic expansion. |
Negative Societal Impacts
Despite the potential benefits, the implementation of Super Power Break Technology also presents potential negative consequences that need to be carefully considered and mitigated. These concerns range from weaponization to environmental hazards.
Negative Impact | Description | Example |
---|---|---|
Weaponization | The technology’s ability to break down materials could be misused in the creation of advanced weaponry, potentially leading to an escalation of conflicts and increased global instability. | The technology could be used to create highly destructive weapons by efficiently breaking down materials to create novel explosives or other destructive devices. Strict regulations and international cooperation would be crucial to prevent such misuse. |
Environmental Concerns | While the technology aims to improve sustainability, improper implementation or unforeseen consequences could lead to new environmental challenges. Careful environmental impact assessments are crucial. | If the process of breaking down materials generates harmful byproducts or releases pollutants into the environment, it could counteract the intended positive environmental impacts. Rigorous environmental monitoring and control measures would be essential. |
Unequal Access | The benefits of Super Power Break Technology may not be equally distributed, leading to social and economic disparities. Efforts must be made to ensure equitable access and prevent the exacerbation of existing inequalities. | The high cost of implementation or specialized knowledge required to utilize the technology could limit access for certain communities or countries, creating a technological divide and worsening existing inequalities. |
Economic Considerations
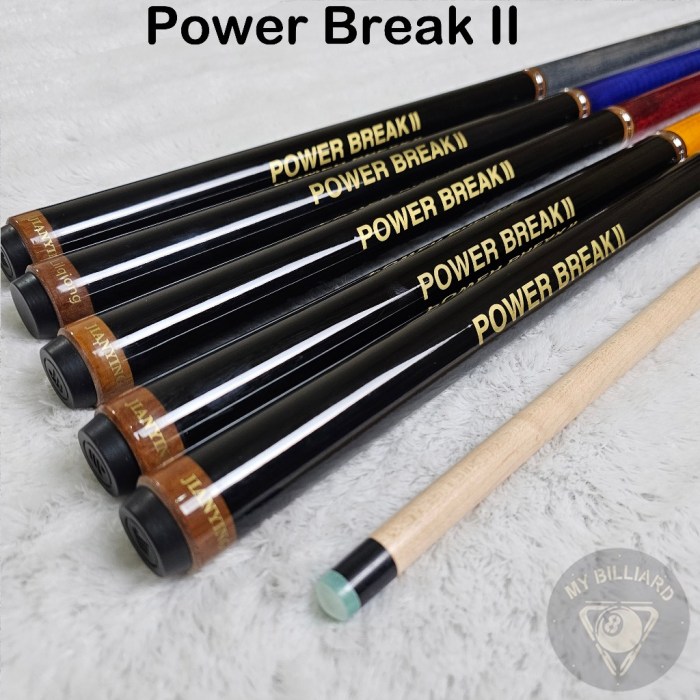
The economic implications of Super Power Break Technology (SPBT) are multifaceted, encompassing substantial initial investment, potential for significant returns, and the possibility of both job creation and displacement across various sectors. A comprehensive economic model must consider the interplay of research costs, manufacturing expenses, market demand, and societal adaptation to this transformative technology.
The potential market for SPBT is vast, extending across numerous industries. A hypothetical model could segment the market based on application: transportation (high-speed rail, aviation), energy (efficient energy storage and transmission), construction (enhanced building materials and infrastructure), and defense (advanced weaponry and protective systems). Each segment presents unique market sizes and growth trajectories, influenced by factors like technological maturity, regulatory frameworks, and consumer adoption rates. For instance, the initial market penetration might be strongest in niche sectors like high-speed rail, where the economic benefits of reduced travel times and increased efficiency are readily apparent. Subsequently, broader adoption in other sectors would depend on cost reduction, improved performance, and overcoming potential regulatory hurdles.
Market Size and Growth Projections
This section details projected market size and growth for SPBT across various sectors. We can model this using a bottom-up approach, estimating the potential market value within each segment (e.g., transportation, energy) based on anticipated adoption rates and the price point of SPBT-enabled products and services. For example, if we project that 10% of high-speed rail lines globally adopt SPBT within the first five years of its commercial availability, and the average cost savings per kilometer of track are $1 million, the total market value within that segment alone would be substantial. Similarly, estimations can be made for other sectors based on comparable data and projected adoption rates. The model would incorporate factors like technological advancements, competitive landscape, and economic growth to forecast future market expansion.
Research, Development, and Deployment Costs
The development and deployment of SPBT will require substantial upfront investment. Research and development (R&D) costs would include personnel expenses for scientists and engineers, laboratory facilities, and specialized equipment. The cost could be estimated based on comparable large-scale technological projects, such as the development of the Human Genome Project or the International Space Station. These projects serve as benchmarks for the scale of investment required for groundbreaking scientific endeavors. Furthermore, the costs of manufacturing and deployment will vary depending on the scale of production and the specific application. Initial production runs will likely be expensive due to low economies of scale, but these costs are expected to decrease as production volume increases. The overall cost structure would include material costs, manufacturing infrastructure, distribution, and installation.
Job Creation and Economic Disruption
SPBT’s introduction will undoubtedly cause both job creation and displacement. New jobs will be created in research, development, manufacturing, installation, maintenance, and related service sectors. The extent of job creation will depend on the speed of adoption and the scale of the technology’s deployment. However, it’s equally important to consider potential job displacement in sectors that become obsolete or significantly altered by SPBT. For instance, certain roles in the transportation sector (e.g., traditional train braking systems maintenance) may become redundant. To mitigate this disruption, proactive measures like retraining and upskilling programs will be crucial to ensure a smooth transition for affected workers and to maintain social stability. Furthermore, government policies could play a significant role in managing the economic impact, fostering innovation, and promoting equitable distribution of benefits and costs.
Ethical Implications
The development and deployment of super power break technology present a complex web of ethical dilemmas, demanding careful consideration of potential consequences across various societal sectors. The power to drastically alter or even halt technological advancements carries immense responsibility, requiring a proactive and nuanced approach to mitigate potential harms and maximize benefits.
The ethical considerations surrounding super power break technology are multifaceted, encompassing issues of individual liberty, societal progress, and global equity. A thorough ethical assessment necessitates the application of various frameworks to navigate the intricate moral landscape presented by this powerful technology.
Ethical Frameworks for Super Power Break Technology
Several ethical frameworks can be applied to analyze the ethical implications of super power break technology. Utilitarianism, for example, would focus on maximizing overall societal well-being by weighing the potential benefits against potential harms. Deontology, on the other hand, would emphasize the inherent rights and duties involved, focusing on whether the technology respects fundamental human rights regardless of its consequences. Virtue ethics would examine the character and motivations of those developing and deploying the technology, emphasizing the importance of responsibility and prudence. Applying these diverse frameworks allows for a more comprehensive ethical evaluation.
Potential for Misuse and Abuse
The potential for misuse and abuse of super power break technology is a significant ethical concern. A powerful tool capable of halting technological progress could be wielded by authoritarian regimes to suppress dissent, stifle innovation, or maintain control. Similarly, powerful corporations might utilize it to protect their market dominance, hindering competition and innovation. Safeguards against such misuse are crucial, requiring robust regulatory frameworks and international cooperation to prevent its application for malicious purposes. For instance, a hypothetical scenario could involve a nation-state halting the development of renewable energy technologies to maintain its dependence on fossil fuels.
Impact on Scientific Advancement and Innovation
The application of super power break technology raises questions about its impact on scientific progress and technological innovation. While it could prevent the development of harmful technologies, it also risks stifling beneficial advancements. A delicate balance must be struck, ensuring that the potential harms of a particular technology are sufficiently significant to warrant its suppression while also protecting the freedom of scientific inquiry and innovation. The potential chilling effect on research and development must be carefully considered and mitigated through transparent and accountable decision-making processes. This requires a nuanced approach that considers both short-term and long-term consequences. For example, prematurely halting research on artificial intelligence could inadvertently impede advancements in medical diagnosis and personalized medicine.
Global Equity and Access
The development and deployment of super power break technology necessitate careful consideration of global equity and access. Unequal access to this technology could exacerbate existing inequalities, allowing powerful nations or corporations to control technological advancement to their advantage. International cooperation and equitable distribution of decision-making power are essential to ensure that this technology is used for the benefit of all humanity, not just a select few. A global governance structure may be required to prevent its use to widen the gap between developed and developing nations, ensuring a fair and just distribution of its benefits.
Energy Sources and Efficiency
The effectiveness of super power break technology hinges critically on its energy source and the efficiency with which that energy is harnessed and utilized. A system requiring exorbitant energy input would be impractical, environmentally damaging, and likely economically unviable. Therefore, careful consideration of energy sources and their associated efficiencies is paramount.
Powering a system capable of generating such significant braking force requires a robust and reliable energy source. Several options exist, each with its own advantages and disadvantages concerning energy density, environmental impact, and cost.
Potential Energy Sources for Super Power Break Technology
Several energy sources could potentially power super power break technology. These include high-capacity batteries (such as lithium-ion or solid-state batteries), supercapacitors, and even hybrid systems combining multiple sources. The selection depends on factors like the required power output, the duration of braking events, the weight constraints of the system, and the overall cost. For instance, high-performance electric vehicles already utilize sophisticated battery systems capable of delivering significant power for acceleration and braking regeneration. Adapting and scaling these systems could provide a viable pathway for super power break technology. Supercapacitors offer rapid charge and discharge capabilities, making them ideal for applications requiring short bursts of high power, while a hybrid system could leverage the strengths of both batteries and supercapacitors for optimal performance.
Energy Efficiency Comparison of Potential Power Sources
A comparative analysis of the energy efficiency of different power sources reveals significant variations. Lithium-ion batteries currently achieve energy densities around 150-250 Wh/kg, while solid-state batteries promise higher densities (potentially exceeding 400 Wh/kg) but are still under development. Supercapacitors, on the other hand, boast extremely high power densities but lower energy densities compared to batteries. Their efficiency in energy storage and release is typically higher than batteries, often exceeding 90%, but their overall energy storage capacity is lower. The energy efficiency of a given system also depends on the conversion efficiency of the energy into braking force. Losses can occur through heat generation in components like motors and actuators. For example, regenerative braking systems in electric vehicles already demonstrate significant efficiency improvements compared to traditional friction-based braking systems, often recovering 20-30% of kinetic energy. However, losses still occur due to electrical resistance and other factors.
Energy Efficiency and Overall System Effectiveness
The energy efficiency directly impacts the overall effectiveness of the super power break technology. A system with high energy efficiency will require less energy input to achieve the same braking performance, leading to several benefits. This translates to longer operational times for battery-powered systems, reduced environmental impact through lower energy consumption, and potentially lower operational costs. Moreover, higher energy efficiency allows for a smaller, lighter system, which is crucial for mobile applications such as vehicles. For instance, a system with 80% efficiency will require 20% less energy than a 60% efficient system for equivalent braking power, resulting in significant advantages in terms of weight, size, and cost. This highlights the importance of optimizing every aspect of the energy conversion and utilization process to maximize the overall effectiveness of the super power break technology.
Safety and Security Protocols
The implementation of Super Power Break Technology necessitates a robust and comprehensive safety and security framework. This framework must account for all phases of the technology’s lifecycle, from design and manufacturing to operation and decommissioning, mitigating potential risks to both personnel and the environment. A multi-layered approach, incorporating both preventative and reactive measures, is crucial for ensuring the safe and responsible use of this powerful technology.
Operational safety protocols must prioritize the prevention of accidental activation and uncontrolled energy release. This requires rigorous testing and validation of all system components, including fail-safe mechanisms and emergency shutdown procedures. Regular maintenance and inspection schedules are essential to detect and address potential issues before they escalate into safety hazards. Furthermore, clear and concise operational guidelines, coupled with comprehensive training for personnel, are vital for ensuring adherence to safety standards.
Operational Safety Protocols
Operational safety protocols encompass a range of procedures designed to minimize the risk of accidents during the technology’s operation. These protocols include, but are not limited to, strict access control to operational areas, mandatory use of personal protective equipment (PPE), regular system diagnostics and performance monitoring, and clearly defined emergency response plans. The implementation of a sophisticated monitoring system, capable of detecting anomalies and triggering automated safety responses, is also paramount. For example, sensors could detect unusual energy fluctuations or system malfunctions, initiating an immediate shutdown sequence. This system would require regular calibration and testing to maintain its effectiveness. Detailed emergency procedures, including evacuation protocols and communication strategies, must be established and regularly practiced.
Security Vulnerabilities and Mitigation Strategies
The advanced nature of Super Power Break Technology presents potential security vulnerabilities that must be addressed proactively. These vulnerabilities could range from unauthorized access and control to malicious attacks aimed at disrupting or weaponizing the technology. A multi-layered security approach is essential, incorporating both physical and cyber security measures.
- Unauthorized Access: Mitigation strategies include biometric authentication, multi-factor authentication, and restricted physical access to operational areas. Regular security audits and penetration testing are crucial to identify and address potential weaknesses.
- Cyberattacks: Robust cybersecurity measures, such as intrusion detection and prevention systems, firewalls, and regular software updates, are necessary to protect the technology from cyber threats. Data encryption and secure communication protocols are also essential to protect sensitive information.
- Physical Tampering: Mitigation strategies include tamper-evident seals, surveillance systems, and robust physical security measures to deter and detect unauthorized access or tampering.
Fail-Safe Mechanisms and Redundancy
The incorporation of robust fail-safe mechanisms and redundancy is crucial for ensuring the safe operation of Super Power Break Technology. Fail-safe mechanisms are designed to prevent catastrophic failures by automatically shutting down the system in the event of a malfunction. Redundancy involves incorporating backup systems that can take over if a primary system fails. For example, a dual power supply system with automatic failover would ensure continuous operation even if one power source is compromised. Similarly, multiple independent sensors could monitor critical parameters, providing redundancy and increased reliability. Regular testing and validation of these mechanisms are vital to ensure their effectiveness. The design should incorporate a layered approach to redundancy, ensuring that multiple layers of protection are in place to mitigate the risk of cascading failures.
Applications and Use Cases
Super Power Break Technology (SPBT), with its ability to instantaneously halt and precisely control kinetic energy, offers a transformative potential across numerous sectors. Its applications extend beyond simple braking systems, impacting fields requiring precise energy management and control. The following examples illustrate the diverse and impactful uses of this revolutionary technology.
The versatility of SPBT stems from its ability to not only stop motion but also to precisely regulate energy transfer. This allows for applications far beyond traditional braking systems, opening doors to innovations across diverse sectors.
Super power break technology is rapidly advancing, driven by innovations in materials science and electronics. A key player in this field, providing crucial memory and storage solutions for these advanced braking systems, is micron technology inc. Their contributions are vital to the development of increasingly responsive and reliable braking systems for both consumer and industrial applications, ensuring that super power break technology continues to improve safety and performance.
Transportation Applications, Super power break technology
SPBT’s most immediate application lies within the transportation sector. Imagine vehicles equipped with SPBT systems capable of instantaneous stops, eliminating the need for traditional braking mechanisms and significantly reducing stopping distances. This would dramatically improve road safety, particularly in emergency situations. Furthermore, the precise energy control offered by SPBT could be integrated into advanced suspension systems, providing superior comfort and handling. High-speed rail systems could benefit from the increased safety and efficiency offered by SPBT, enabling higher speeds and shorter travel times. Finally, SPBT could revolutionize aerospace braking systems, enabling safer and more controlled landings for aircraft and spacecraft.
Industrial Applications
In industrial settings, SPBT could revolutionize safety protocols. Heavy machinery, such as cranes and robotic arms, could be equipped with SPBT to prevent catastrophic accidents caused by uncontrolled movement. The precise energy control allows for smoother, more efficient operations, reducing wear and tear on equipment and minimizing energy waste. Furthermore, SPBT could enhance the safety of manufacturing processes involving high-speed machinery, minimizing the risk of injuries and damage. Precise control over kinetic energy could also optimize the efficiency of various industrial processes involving moving parts.
Medical Applications
The medical field could benefit significantly from the precise control over kinetic energy offered by SPBT. Imagine surgical robots equipped with SPBT, allowing for incredibly precise movements during delicate procedures. This could lead to less invasive surgeries with faster recovery times and fewer complications. Furthermore, SPBT could improve the safety of medical equipment such as centrifuges and imaging devices, minimizing the risk of malfunctions and accidents. Finally, advancements in SPBT could allow for the development of new medical devices and therapies requiring extremely precise control over energy.
Energy Sector Applications
SPBT could play a crucial role in enhancing the efficiency and safety of energy generation and distribution. For example, SPBT could be integrated into wind turbines to rapidly halt blade rotation during emergencies, preventing damage and ensuring the safety of personnel. In hydroelectric power plants, SPBT could provide more precise control over water flow, optimizing energy generation and minimizing environmental impact. Moreover, SPBT could potentially be used in advanced energy storage systems, enabling faster and more efficient charging and discharging cycles.
Disaster Response and Mitigation
SPBT’s capacity for rapid kinetic energy control could prove invaluable in disaster response and mitigation efforts. For example, SPBT could be used to rapidly stop runaway vehicles or other dangerous objects, preventing further damage and injury. Furthermore, SPBT could be integrated into specialized equipment for search and rescue operations, allowing for more precise and controlled movements in challenging environments. In earthquake-prone regions, SPBT could potentially be used to mitigate the impact of seismic activity on structures, reducing the risk of collapse.
Future Applications
As SPBT technology advances, its applications are likely to expand even further. The development of smaller, more efficient SPBT systems could lead to widespread integration into everyday devices, improving safety and efficiency across various aspects of life. Furthermore, advancements in materials science and nanotechnology could enable the creation of SPBT systems with even greater precision and control, opening up entirely new possibilities in fields such as micro-robotics and advanced manufacturing. The integration of SPBT with artificial intelligence could lead to autonomous systems capable of adapting to changing conditions and making real-time decisions regarding kinetic energy management.
Materials Science and Engineering
The development of Super Power Break Technology hinges critically on advancements in materials science. The extreme forces and energy densities involved demand materials possessing exceptional properties far beyond those currently available in many applications. The selection and engineering of these materials will directly influence the technology’s efficiency, safety, and overall feasibility.
The successful implementation of Super Power Break Technology necessitates materials with a unique combination of properties. These materials must exhibit high strength-to-weight ratios to minimize system mass and improve overall performance. Furthermore, they need to possess exceptional thermal conductivity to efficiently dissipate the significant heat generated during braking. Resistance to extreme temperatures, pressures, and wear is also crucial to ensure the longevity and reliability of the system. Finally, the materials must be compatible with the chosen energy storage and conversion mechanisms.
Material Properties for Super Power Break Systems
High-strength, lightweight materials like carbon fiber composites or advanced alloys (e.g., titanium alloys, nickel-based superalloys) are prime candidates for structural components. These materials offer a balance between strength and low density, crucial for minimizing the overall weight of the braking system and maximizing its efficiency. High thermal conductivity materials such as diamond, silicon carbide, or aluminum nitride are essential for efficient heat dissipation. The ability to rapidly and effectively transfer heat away from the braking surfaces prevents overheating and failure. Materials with high wear resistance, such as ceramics or advanced metal matrix composites, are needed to withstand the frictional forces generated during high-energy braking events. This reduces the need for frequent maintenance and replacement. The selection of specific materials will depend on the specific application and operating conditions of the Super Power Break Technology. For instance, aerospace applications might prioritize lightweight materials with exceptional high-temperature resistance, while ground-based applications might favor materials with superior wear resistance.
Material Limitations and Research Needs
Despite significant advancements, current materials still face limitations in meeting the extreme demands of Super Power Break Technology. For instance, many high-strength materials are brittle and susceptible to cracking under high stress, requiring careful design considerations to mitigate these risks. The high temperatures generated during braking can also degrade the performance of some materials over time, necessitating the development of materials with enhanced thermal stability and resistance to degradation. Further research is needed in developing materials with even higher strength-to-weight ratios, improved thermal conductivity, and enhanced resistance to wear and high-temperature degradation. Exploring novel material processing techniques, such as additive manufacturing or advanced surface treatments, could lead to significant improvements in material properties and performance. Investigating the potential of emerging materials like graphene or carbon nanotubes could also unlock significant breakthroughs in the field. The development of self-healing materials or materials with improved damage tolerance is another promising research direction that could enhance the reliability and lifespan of Super Power Break systems.
Environmental Considerations
The development and deployment of Super Power Break Technology (SPBT) necessitates a thorough assessment of its potential environmental impact, encompassing both the manufacturing process and its operational phase. Minimizing the technology’s ecological footprint is crucial for its responsible and sustainable implementation. This section details the potential environmental effects and proposes mitigation strategies, comparing SPBT’s impact to existing braking technologies.
The manufacturing process of SPBT, depending on the specific materials and techniques employed, could involve significant energy consumption and the generation of waste. For example, the production of advanced composite materials often requires high temperatures and energy-intensive processes, potentially leading to greenhouse gas emissions. The extraction and processing of rare earth elements, if utilized in SPBT components, could also raise concerns about habitat disruption and water pollution. Furthermore, the disposal of worn-out SPBT components needs careful consideration to prevent the release of harmful substances into the environment.
Manufacturing Process Impacts
The environmental impact of SPBT manufacturing will depend heavily on the specific materials used. Using recycled materials, optimizing energy efficiency during production, and implementing robust waste management strategies are vital. For instance, the adoption of closed-loop manufacturing processes can significantly reduce waste generation and resource consumption. Life cycle assessments (LCAs) should be conducted throughout the development process to identify and mitigate potential environmental hotspots. This approach would involve quantifying the environmental burden associated with each stage of the SPBT lifecycle, from raw material extraction to end-of-life disposal. A comparative LCA with existing braking systems will offer a valuable benchmark for evaluating the overall environmental performance of SPBT.
Operational Impacts and Mitigation
During operation, SPBT may generate heat and potentially release small amounts of particulate matter, depending on the specific mechanism. The quantity of these emissions will need to be carefully assessed and compared to existing braking technologies. For example, friction-based braking systems already generate considerable heat and particulate matter, contributing to air pollution, particularly in urban areas. SPBT’s potential for reduced wear and tear on components could lead to a decrease in particulate matter generation compared to traditional braking systems. Efficient thermal management systems, such as advanced cooling mechanisms, are crucial for mitigating the environmental impact of heat generation. Regular maintenance and timely replacement of components will also help minimize environmental effects.
Comparison with Existing Technologies
Compared to traditional friction-based braking systems, SPBT has the potential for reduced wear and tear, translating to less particulate matter emission. However, the energy consumption associated with the manufacturing and operation of SPBT must be carefully considered. A thorough life-cycle assessment will be crucial in determining the overall environmental superiority of SPBT over conventional braking systems. For instance, regenerative braking systems, already present in many electric vehicles, recover kinetic energy during braking, reducing energy consumption and emissions. SPBT, if designed to incorporate regenerative braking principles, could further enhance its environmental profile. The comparison should account for all aspects of the lifecycle, including material extraction, manufacturing, operation, and end-of-life management, providing a comprehensive environmental impact assessment.
Research and Development Roadmap
Developing Super Power Break Technology requires a phased approach, integrating fundamental research with applied engineering and rigorous testing. This roadmap Artikels key milestones and potential challenges in bringing this transformative technology to market. Success hinges on collaborative efforts across diverse scientific disciplines and substantial investment in research infrastructure.
Phase 1: Fundamental Research and Proof of Concept (Years 1-3)
This initial phase focuses on establishing the fundamental scientific principles underpinning Super Power Break Technology. We need to validate theoretical models and demonstrate the feasibility of creating a material with the desired properties.
Key Milestone: Successful demonstration of a laboratory-scale prototype exhibiting a significant improvement in braking performance compared to existing technologies. This will involve achieving a specific target reduction in stopping distance under controlled conditions.
Challenges: Identifying and synthesizing suitable materials with exceptional strength, durability, and energy absorption capabilities. Overcoming potential material limitations such as brittleness or fatigue.
Phase 2: Material Optimization and Scalable Production (Years 4-7)
This phase concentrates on optimizing material properties, developing scalable manufacturing processes, and conducting rigorous testing to ensure reliability and safety. This involves transitioning from laboratory-scale prototypes to larger, more robust models.
Key Milestone: Development of a scalable manufacturing process capable of producing high-quality materials consistently and cost-effectively. This will require refining production techniques to meet specific tolerances and quality standards.
Challenges: Scaling up material production while maintaining consistent quality. Addressing potential manufacturing challenges related to material processing, cost optimization, and waste management.
Phase 3: System Integration and Testing (Years 8-10)
This phase integrates the optimized materials into a functional braking system, followed by comprehensive testing and validation in various environments and conditions. This involves rigorous simulations and real-world testing to ensure safety and performance.
Key Milestone: Successful completion of rigorous testing and validation of the integrated braking system in various real-world scenarios, demonstrating superior performance and reliability compared to current state-of-the-art braking systems. This will include testing under diverse environmental conditions, such as extreme temperatures and high humidity.
Challenges: Integrating the new material into existing vehicle architectures. Ensuring compatibility with existing vehicle control systems. Addressing potential safety concerns and obtaining necessary regulatory approvals.
Phase 4: Commercialization and Deployment (Years 11-15)
The final phase focuses on commercialization, marketing, and deployment of the technology. This includes securing regulatory approvals, establishing manufacturing partnerships, and launching the product into the market.
Key Milestone: Successful launch of Super Power Break Technology into the commercial market, with initial adoption by key vehicle manufacturers. This will involve securing strategic partnerships and establishing a robust supply chain.
Challenges: Competition from established braking technology manufacturers. Educating the public and building trust in the new technology. Addressing potential market barriers and ensuring cost-effectiveness.
Closing Notes: Super Power Break Technology
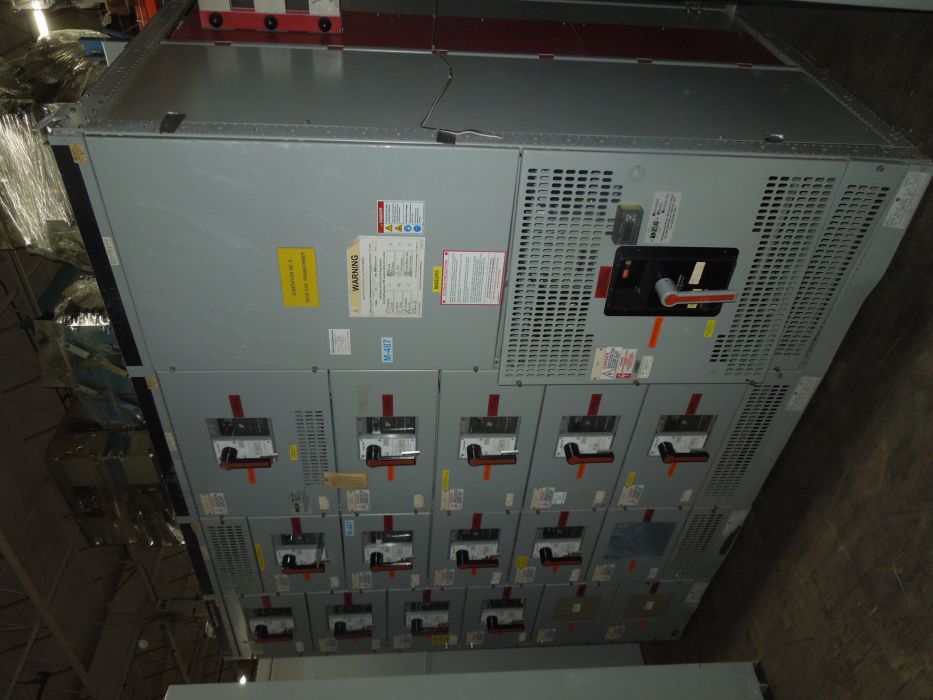
In conclusion, the pursuit of Super Power Break Technology presents both immense potential and considerable challenges. While the societal benefits—from improved infrastructure to novel energy sources—are compelling, the ethical implications and potential for misuse demand careful consideration. A responsible and collaborative approach, encompassing rigorous research, robust safety protocols, and transparent regulatory oversight, will be crucial in navigating the complexities and ensuring that this technology, if realized, serves humanity’s best interests. The road ahead is paved with both exciting possibilities and significant uncertainties, demanding a balanced and informed approach to its development and deployment.
Super power break technology is a fascinating field, constantly pushing the boundaries of what’s possible. The development and implementation of such advanced systems often relies heavily on the expertise of a chief technology officer , who guides the technological vision and strategic direction of the entire company. Ultimately, the success of these groundbreaking power break technologies hinges on strong leadership and innovative engineering.